Microbial colonization as a determinant factor for health during the first 1000 days of life
By Pr. Marie-Claire Arrieta
Departments of Physiology and Pharmacology & Pediatrics, Cumming School of Medicine, University of Calgary, Health Research Innovation Centre, Calgary, Alberta, Canada
Sources
This article is based on scientific information
Sections
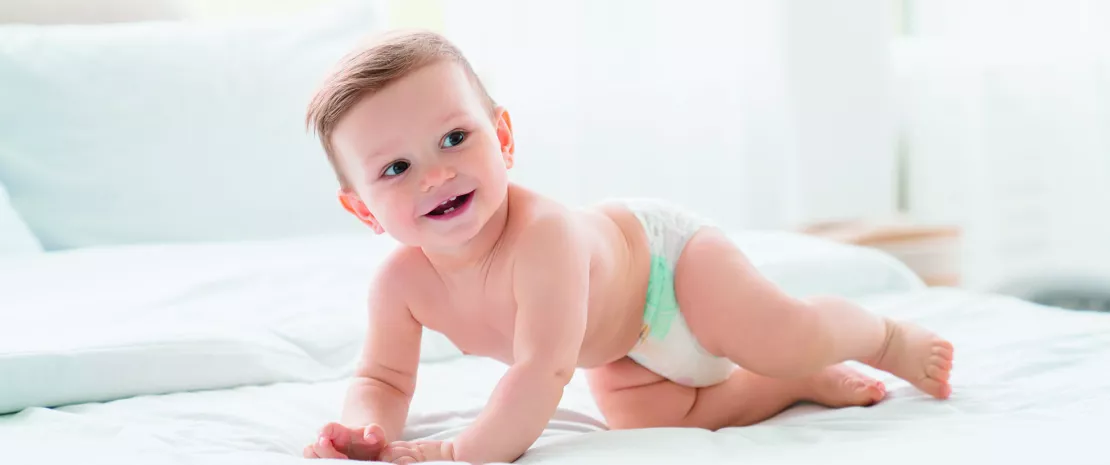
About this article
Epidemiological and mechanistic studies during the past 20 years have demonstrated a role for the early-life microbiome in the pathogenesis of several non-communicable diseases (NCDs). This collection of trillions of microorganisms, residing mainly in the intestines, engages in a dynamic crosstalk with host cells. It is through this crosstalk that the host integrates microbial metabolites and structures to the early programming of immune, neurologic, metabolic and endocrine mechanisms that support host development. While this crosstalk occurs throughout life, there is a non‐redundant period in early development, known as a “window of opportunity”, during which microbial-host crosstalk sets the stage for host homeostasis, or deviations from it. This period lasts approximately 1,000 days, encompassing fetal growth and the first two years of human life, and it is at the center of intense investigation.
COMPOSITION AND FUNCTION OF THE EARLY-LIFE MICROBIOME
The infant gut microbiome starts developing at birth as a very simple ecosystem, gaining species diversity for about 2-3 years (box 1). This process occurs in a stepwise fashion, with common patterns identified across several human populations (Figure 1). Early colonization starts with pioneer species that primarily originate from the vaginal canal and maternal feces or the skin, depending on whether the infant is delivered vaginally or by Cesarean (C)-section, respectively. Vaginally-born exhibit higher abundance in Lactobacillus, Prevotella, and Sneathia, while those born by C-section are initially colonized by Staphylococcus, Propionibacterium, and Corynebacterium. Breastfed infants exhibit increased abundance of Bifidobacterium sp. and Lactobacillus sp., compared to formula fed infants, which display increased abundance of Bacteroides, Enterobacteriaceae and Clostridiaceae. As solid foods are introduced, the gut microbiome becomes increasingly diverse and shifts towards a state of Bacteroidaceae, Lachnospiraceae, and Ruminococcaceae dominance that persists into adulthood (Figure 1) [1].
The infant gut is stage of an important metabolism that contributes to digestion, energy metabolism and immune education. Through microbial digestion of breastmilk components, Bifidobacterium species decrease the intestinal luminal pH through the production of lactate and acetate, which is considered a crucial strategy in increasing intestinal nutrient absorption. Acetate accounts for the majority of the short-chain fatty acids (SCFA) produced in the infant gut, and is involved in preventing infections with enteropathogens [2]. Bifidobacteria are also involved in a process known as cross-feeding, in which the production of acetate and lactate serves as substrates for the growth of other species, such as Roseburia, Eubacterium, Faecalibacterium, and Anaeroestipes, favoring microbiome diversity. Bacteroides species can also ferment breastmilk, and are important producers of the SCFA propionate. Bacteroides species have a unique capacity to also metabolize mucin-derived oligosaccharides [3]. This metabolic plasticity improves their adaptability to the fluctuating intestinal conditions between meals, as well after weaning and introduction of solid foods. Bacteroides species are also key for immune education, constituting an important source of the microbial component lipopolysaccharide, as well as prompting for the development of tolerogenic adaptive immune responses in the gut [4]. Given their special adaptability to the infant gut environment, their demonstrated mother-to-infant strain transmission, their dominance in the infant gut, their importance to other members of this microbial ecosystem, and the benefits to the host, both Bacteroides spp. and Bifidobacterium spp. are likely keystone species of the human infant microbiome (Figure 2).
FACTORS THAT SUSTAIN THE EARLY-LIFE MICROBIOME
Early pioneer species can have long-lasting consequences to the trajectory of the infant gut microbiome through priority effects. This ecological process dictates that early arrival to a new ecosystem plays a fundamental role in the assembly of the community. This process explains the influence of mode of birth on the initial composition of the infant microbiome. Large cohort studies have identified microbiome differences linked to C-section delivery that last for months after birth, likely impacting this critical period in host development [5]. These include a lower of abundance of Bacteroides and Bifidobacterium spp., as well as an increased abundance of potential pathogenic species.
Besides mode of birth, the availability and abundance of nutritional substrates imposes a determinant effect on the early-life microbiome. Breastmilk contains more than 10 g/L of human milk oligosaccharides (HMOs), with 2’fucosyl lactose (2’FL) and trifucosyllacto-N-hexaose (TF-LNH) as the most abundant HMOs [6]. The majority of HMOs are digested by bifidobacterial and Bacteroides spp. into SCFAs. Bifidobacteria have a large repertoire of genes for the digestion of HMOs. Several subspecies of B. longum are commonly found in the infant gut, with B. longum subsp. infantis (B. infantis), B. longum subsp. longum (B. longum), and B. longum subsp. breve (B. breve) commonly isolated from healthy breastfed infant feces, and formula-fed infants often colonized with B. adolescentis. Of these subspecies, B. infantis has the largest gene repertoire to digest all HMO structures in human milk [7]. Breastmilk also influences the composition of the infant microbiome through immune factors, such as antimicrobial compounds (lactoferrin and lysozyme), as well as immune effectors (sIgA, immune cells, and cytokines), which are critical for the immune exclusion of pathogenic microbes [1]. Notably, the lower abundance of Bifidobacterium in formula-fed babies is associated with a lower concentration of lactate, sIgA, and a higher gut luminal pH compared to breastfed babies.
Besides mode of birth and infant nutrition, other factors, such as maternal smoking, maternal body mass index, gestational diabetes, familial asthma and stress can influence the early-life microbiome [8]. The mechanisms underlying the associations between these factors and the infant microbiome remain unclear, but likely involve changes to the maternal microbiome and subsequent vertical transmission to the infant, as well as the increased risk of C-section rate and reduced success in breastfeeding linked to many of these factors. In general, the individual effects of factors such as birth mode, antibiotic use, and breastfeeding are relatively well characterized. However, the combinatory effects of these exposures remain poorly understood.
Is the infant colonized in utero?
- Microbial DNA has been detected in the placenta, amniotic fluid and meconium, prompting for the speculation of in utero colonization.
- Failure to culture microbes detected in utero, the consistent effect of mode of birth on the microbiome, and the successful generation of germ-free animals from embryos have led to the current consensus that healthy newborns start microbial colonization at birth [15].
EARLY-LIFE DYSBIOSIS AS A CAUSE OF NONCOMMUNICABLE DISEASES
As a young ecosystem, the early-life microbiome is inherently less resilient. Ecological resilience refers to the capacity of an ecosystem to revert back to its original state after a perturbance occurs. This places the infant microbiome at a higher risk of permanently altering its trajectory during a critical developmental stage. Peri- and post-natal antibiotic use induce drastic compositional and diversity shifts to the infant microbiome, known as dysbiosis, decreasing the abundance of bifidobacteria and overall microbiome diversity, and increasing pathogenic species. This effect is observed even when antibiotics are only given to the mothers during vaginal birth (to prevent B-Streptococcus infections), and is augmented when given to infants during the first year of life, in a dose response manner [9]. Notably, even a single course of amoxicillin to infants decreased bifidobacterial abundance for several months, highlighting the susceptibility of this important group of bacteria to these commonly used drugs [10].
Antibiotic exposure during gestation or in the pre-weaning stage of rodents can exacerbate allergic immune responses (IgE, Th2 and Th17 lymphocytes), adiposity and obesity, autoimmune responses, and chronic colitis [1]. These systemic responses to early-life dysbiosis are in line with consistent epidemiological findings associating early-life antibiotic use with several NCDs. For example, a systematic review and metanalysis of 13 studies identified a dose-response association between antibiotic use and obesity, which ranged between an 11% increased risk for infants receiving only one dose, to a 24% increased risk with more than one treatment [9]. More recently, a systematic review and metanalysis of 160 studies, encompassing over 22 million children, revealed significant associations between pediatric antibiotic use and atopic dermatitis, food allergies, allergic rhinoconjuntivitis, asthma, juvenile arthritis, psoriasis and autism spectrum disorder [11].
Establishing directionality and causality from epidemiological studies is very difficult. However, the combined results of preclinical studies, with the consistent and dose-response associations between antibiotic use with asthma and obesity, in particular, support for the application of more strict antibiotic stewardship measures. A recent study in Canadian children reported a parallel decrease in asthma incidence as antibiotic prescriptions decreased at the population level between the years 2000 and 2014. Importantly, the microbiome composition at 1 year of age mediated the association between antibiotic exposure and asthma diagnosis at five years [12]. This important study provides strong evidence for a causal relationship between antibiotic use and asthma in humans and reveals the need for a prudent antibiotic use as a strategy to reduce asthma rates.
RESTORING DYSBIOSIS – ARE WE THERE YET?
The detrimental consequences of early-life dysbiosis warrants further study, but also action. Decreasing C-section rates, formula feeding and antibiotic prescriptions, while a worthy goal, have limited potential as successful strategies given societal needs. Several avenues of microbiome restoration have been attempted, with mixed results. Two methods of ecosystem restoration have been tested in planned C-section deliveries: vaginal seeding and fecal microbiota transplantation (FMT). Vaginal seeding involves impregnating the skin and/or oral cavity of a newborn with collected maternal vaginal secretions. The three vaginal seeding trials currently published showed that this method does not restore the C-section microbiota into one resembling that of a vaginal birth [8]. In contrast, a mother-to-infant FMT (given once during the first feed) was sufficient to correct the C-section microbiome [13]. However, while authors carried out pathogen screening in the FMT samples, this controversial practice carries significant and unnecessary infectious risk to an otherwise healthy newborn infant, and it is unlikely to become a viable option.
The use of pre- and probiotics may provide a more practical and feasible approach to microbiome restoration, especially when informed from the studies summarized above. A recent study showed that depletion of bifidobacteria and HMO-utilizing genes could be ameliorated through the combination of administration of a strain of B. infantis and breast feeding [14]. This strategy also dampened pro-inflammatory responses conducive to allergy at 1-year, showing long-term beneficial immune mechanisms. Still, there is insufficient evidence that current microbiome restoration strategies will help curve the alarming rates of pediatric NCDs.
Conclusion
The early-life microbiome is an integral component of child health. Our knowledge on the compositional and functional patterns of early colonization, as well as the factors sustaining or perturbing these patterns has grown considerably. In contrast, the mechanisms that explain how dysbiosis contributes to disease pathogenesis are poorly understood. Ecologicallyinformed measures to replenish keystone species of the infant microbiome lost through antibiotic use, C-section of formula feeding, as well as their nutritional substrates, may prove as effective measures. However, current microbiome restoration strategies are insufficient, and have yet to show effectivity in reducing the risk of NCDs. This is the next crucial step to prompt changes in medical policies and practices.
1. Laforest-Lapointe I, Arrieta MC. Patterns of Early-Life Gut Microbial Colonization during Human Immune Development: An Ecological Perspective. Front Immunol 2017; 8: 788.
2. Fukuda S, Toh H, Hase K, et al. Bifidobacteria can protect from enteropathogenic infection through production of acetate. Nature 2011; 469: 543-7.
3. Sonnenburg JL, Xu J, Leip DD, et al. Glycan foraging in vivo by an intestine-adapted bacterial symbiont. Science 2005; 307: 1955-9.
4. Telesford KM, Yan W, Ochoa-Reparaz J, et al. A commensal symbiotic factor derived from Bacteroides fragilis promotes human CD39(+)Foxp3(+) T cells and Treg function. Gut Microbes 2015; 6: 234-42.
5. Penders J, Thijs C, Vink C, et al. Factors influencing the composition of the intestinal microbiota in early infancy. Pediatrics 2006; 118: 511-21.
6. Thurl S, Munzert M, Boehm G, et al. Systematic review of the concentrations of oligosaccharides in human milk. Nutr Rev 2017; 75: 920-33.
7. Underwood MA, German JB, Lebrilla CB, et al. Bifidobacterium longum subspecies infantis: champion colonizer of the infant gut. Pediatr Res 2015; 77: 229-35.
8. Korpela K, de Vos WM. Infant gut microbiota restoration: state of the art. Gut Microbes 2022; 14: 2118811.
9. Mc- Donnell L, Gilkes A, Ashworth M, et al. Association between antibiotics and gut microbiome dysbiosis in children: systematic review and meta-analysis. Gut Microbes 2021; 13: 1-18.
10. Korpela K, Salonen A, Saxen H, et al. Antibiotics in early life associate with specific gut microbiota signatures in a prospective longitudinal infant cohort. Pediatr Res 2020; 88: 438-43.
11. Duong QA, Pittet LF, Curtis N, et al. Antibiotic exposure and adverse long-term health outcomes in children: A systematic review and meta-analysis. J Infect 2022; 85: 213-300.
12. Patrick DM, Sbihi H, Dai DLY, et al. Decreasing antibiotic use, the gut microbiota, and asthma incidence in children: evidence from population-based and prospective cohort studies. Lancet Respir Med 2020; 8: 1094-105.
13. Korpela K, Helve O, Kolho KL, et al. Maternal Fecal Microbiota Transplantation in Cesarean-Born Infants Rapidly Restores Normal Gut Microbial Development: A Proof-of-Concept Study. Cell 2020; 183: 324-34.e5.
14. Henrick BM, Rodriguez L, Lakshmikanth T, et al. Bifidobacteria-mediated immune system imprinting early in life. Cell 2021; 184: 3884-98.e11.
15. Perez-Muñoz ME, Arrieta MC, Ramer-Tait AE, et al. A critical assessment of the “sterile womb” and “in utero colonization” hypotheses: implications for research on the pioneer infant microbiome. Microbiome 2017; 5: 48.